Methods & Algorithms
Methods & Algorithms
One of the main obstacles for the spectroscopy of biological tissue is the light scattering which might dominate the transport of photons in tissue. The spectroscopic methods applied are:
- cw – spectroscopy in the VIS and NIR – range,
- time - domain spectroscopy with ps – resolution,
- intensity modulated spectroscopy (frequency domain),
- laser – Doppler spectroscopy,
- laser – speckle imaging.
The task is to quantify tissue chromophores, mainly:
- haemoglobin, both in oxygenated and deoxygenated form,
- myoglobin,
- lipid,
- water, and others, as well as
- scattering properties.
The spectroscopic challenge is the separation of absorption from scattering properties of the tissue which might be inhomogeneous and of unknown geometry. For the diffuse transport of photons in tissue mathematical models are used, mainly analytical solutions or statistical methods like Monte Carlo simulations.
We developed spatially-resolved, broad-band spectroscopy for the quantification of tissue absorption coefficients µa(l) and a subsequent calculation of tissue chromophores. In the figure attenuation spectra are shown for different source detector separation with the characteristic signatures of water and haemoglobin. Tissue deoxygenation during cuff occlusion results in changes of the spectra. The µa – spectra on the right are based on an analytical model for the slope in attenuation with respect to the distance. The abundance of measured spectral data allows the underlying hypotheses of the model to be tested.
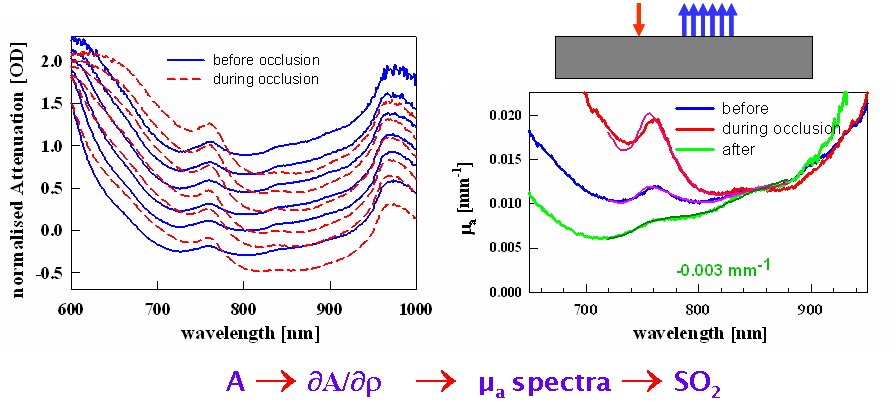
Monte Carlo simulations allow the calculation of the transport of photons in inhomogeneous geometries and give e.g. the reflected intensity as a function of source-detector distance, the photon time-of-flight or the pathlength as a function of tissue depth. A difficulty is that the optical properties of the tissue have to be assumed. In the example the depth sensitivity of a measurement is shown based on Monte Carlo simulations for Modified-Lamber-Beer (MLB) and Spatially-Resolved-Spectroscopy (SRS).
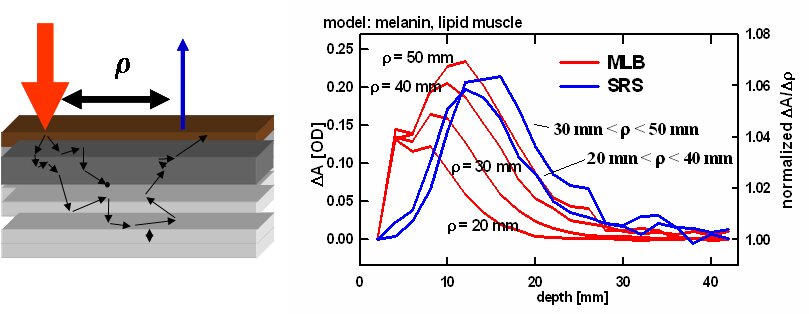
A short laser pulse is temporally spread out in tissue and therefore the measurement of the transit time of the light contains information of the optical pathlength. This can be measured with a system based on time-correlated single photon counting (TCSPC) with picosecond laser pulses in conjunction with a fast detectors and detector electronics. In the figure the laser pulse broadening on a sub-nanosecond time scale depending on the tissue haemoglobin concentration in the arm of a volunteer is shown. Information of the time-spread can be exploited for the calculation of either absolute concentrations of chromophores or for retrieval of depth information.
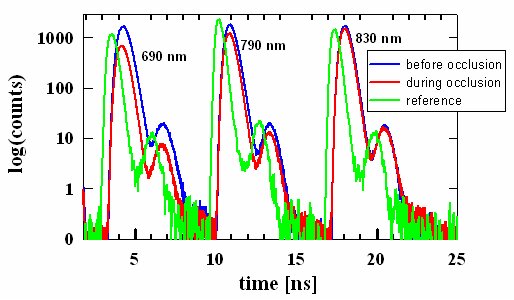
We showed that in cortical tissue absolute haemoglobin concentrations can be derived and combined with blood flow monitoring based on Laser-Doppler spectroscopy. In the figure changes in these parameters for the cortex of a rat is shown following seven short activation bouts.
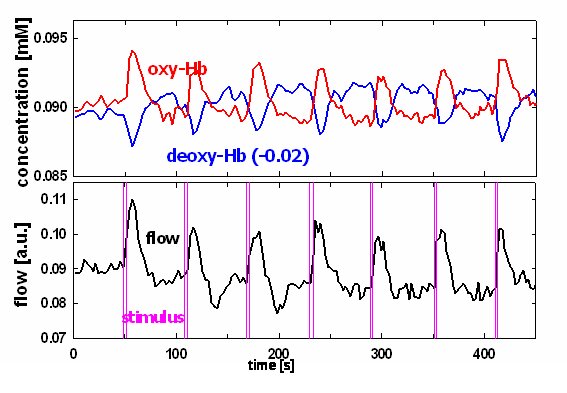
A much disputed issue in neuro-vascular coupling was whether there is an early deoxygenation (‘dip’) following cortical activation, with the initial data coming from optical spectroscopy and large implication for all functional imaging modalities. We showed that the inclusion of a physical model for the wavelength dependent pathlength of photons in cortical tissue significantly changes the calculated haemoglobin traces. In the figure this is demonstrated where the same optical spectra after a stimulation period (green) were analysed. Without model (dashed) there is a significant increase within the first seconds which disappears with the physics based model.
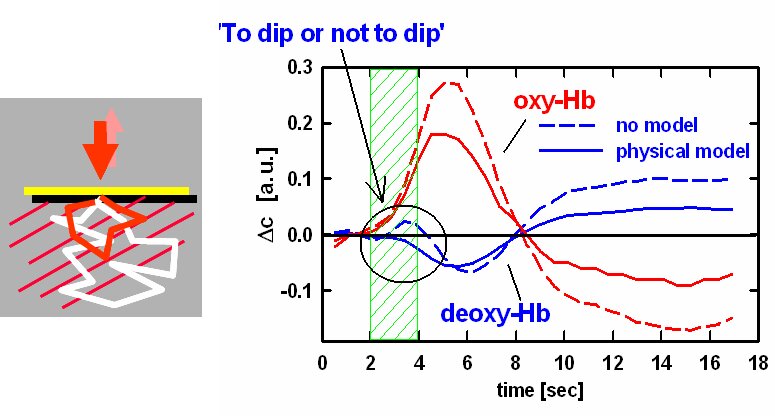
The measurement of body fat and in particular of subcutaneous fat is still unreliable without imaging methods like ultrasound or MRI. We showed that NIRS can be used to determine subcutaneous fat (adipose tissue thickness) by comparison with ultrasound, MRI and calliper. This is based on the lipid absorption peak at wavelengths close to 930 nm. In the lower part of the figure the correlation between ultrasound and NIRS data is shown for 240 measurements on human subjects, giving an estimate of errors of less than 0.25 mm for the lipid layer.
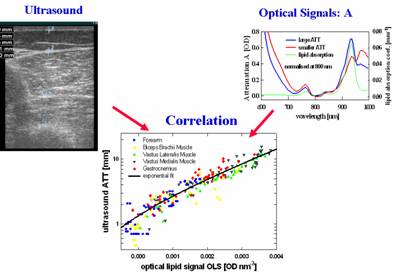
A widely used approach to derive muscle oxygenation data is Spatially-Resolved spectroscopy SRS. The underlying assumption of a homogeneous medium allows analytical equations for the light transport in tissue to be used. However this is flawed when the subcutaneous lipid layer is large. Based on Monte Carlos simulations we developed an algorithm to overcome this limitation.
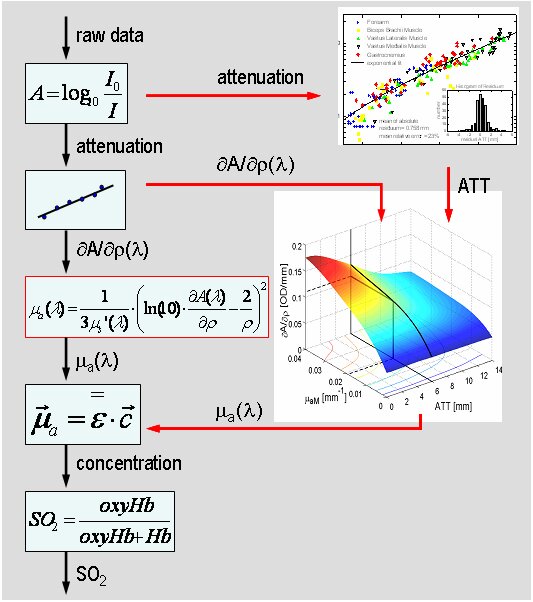
When Laser speckle are imaged with a camera, the speckle are ‘smeared’ out on the detector area depending on both the image acquisition time and the movements of the object. Therefore, in perfused tissue the contrast in images is a related to the blood flow. Based on this method we map changes in blood flow in cortical tissue or skin.
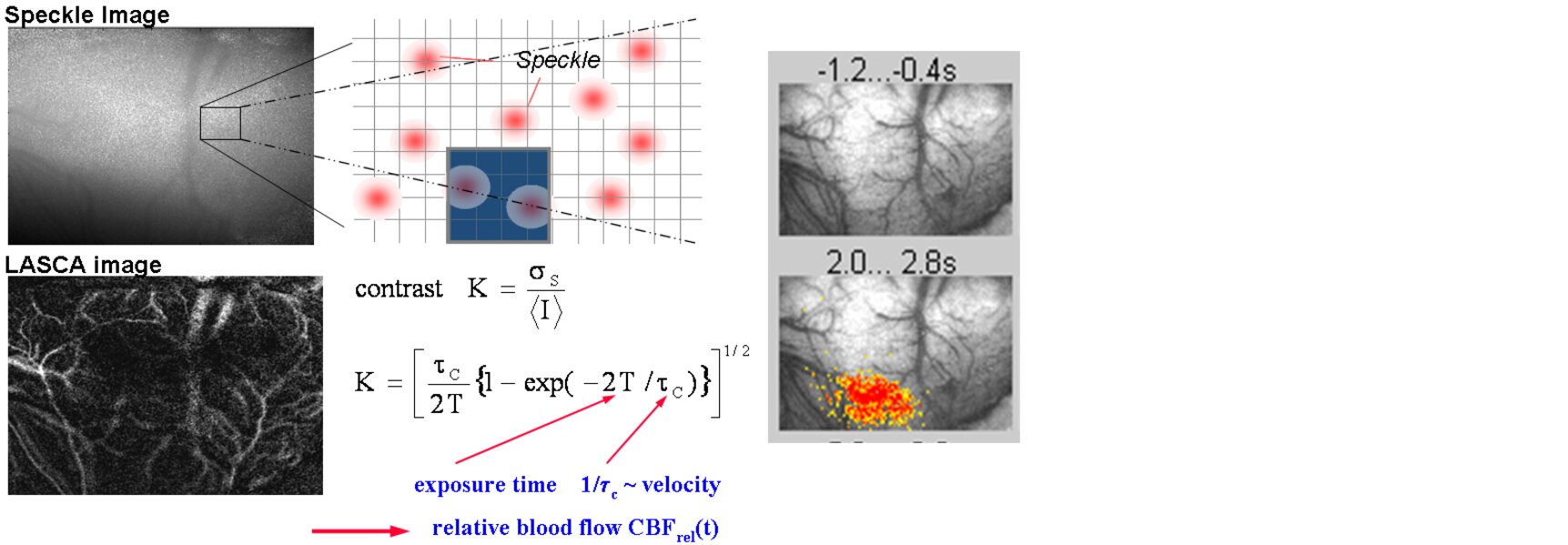
We showed that from spectral measurements of attenuation changes in the concentration of haemoglobin, cytochrome oxidase and of tissue scattering can be separated. This was demonstrated in a rat model during cortical spreading depression, which is linked to stroke and migraine.
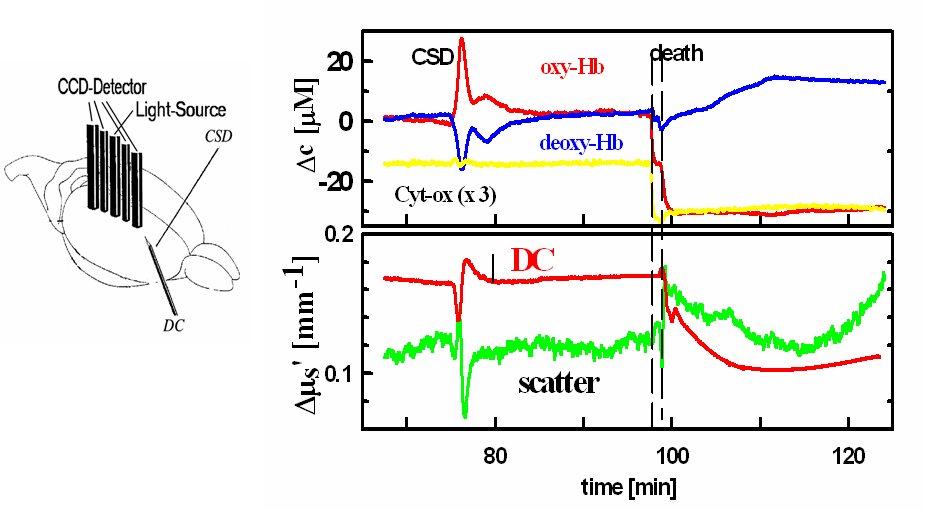